Tennis’ Kinetic Chain: Should You Push or Pull?
DEFINITION OF THE KINETIC CHAIN
The term kinetic chain refers to a specific conceptual framework for understanding the mechanisms by which athletes accomplish the complex tasks required to function in sport. It is a coordinated sequence of activation, mobilization, and stabilization of body segments to generate and regulate force, create movement, and protect tissue from increased stress during athletic activity1.
Kinetic chain sequencing serves three purposes:
- Efficient generation and transfer of kinetic energy and force to the distal segment to move an object1, achieved by using the summation of speed principle, where the speed and force developed in each segment is divided by the actions of the proximal segments is facilitated and reinforced, similar to cracking a whip,
- stabilizing and positioning the body segments and joints to regulate and absorb the forces developed at the joints, which is achieved by creating predictive postural adjustments that fit into the sporting activity pattern are integrated to maintain a stable base for activities2,3,
- Postural stabilization to counteract the eccentric loads and destabilizing effects of athletic movements, accomplished by integrating proximal and distal muscle activation to relieve loads across the distribute the entire limb4, control of eccentric and tension loads, and place the joints in their most stable configuration in the upper or lower limb5
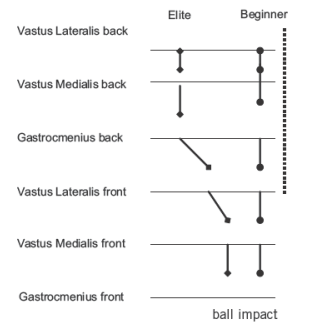
The serve is considered by many to be the most important shot in tennis. It’s a common activity, it can dictate the conditions under which each point is played, and it’s the only shot over which the player has control before hitting the ball. The serve has components like speed, placement, and spin, all of which are related to effectively developing an efficient kinetic chain. This should result in optimal club placement at maximum speed and the desired trajectory to go up and through the ball. To do this, the body must go down and back into a kinematic cocking position and a kinematic loading position, and then the arm must move quickly forward and through the impact of the ball. There are two ways to perform this move: squeeze the body and arm through the impact of the ball, or pull through. These two kinetic chain patterns have observable differences in how the segments are activated and moved, and have different physiological stresses and biomechanical outcomes for impact performance.
KINETIC CHAINS PUSH AND PULL
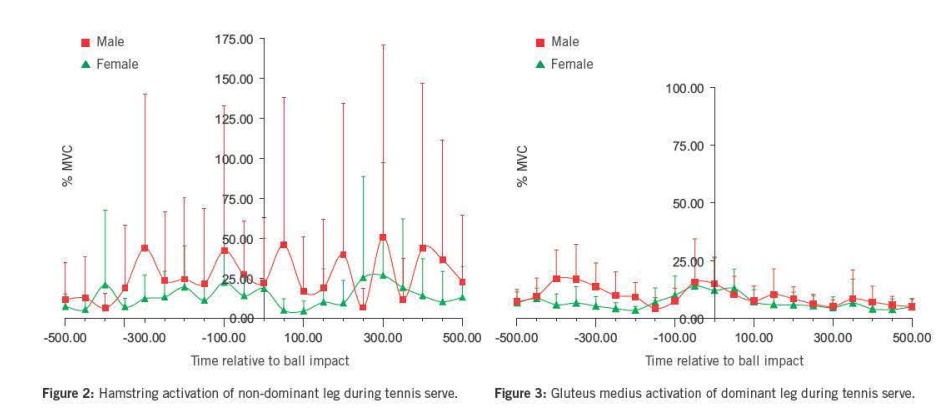
The push through activation sequence uses knee flexion and hind leg drive to maximize ground reaction forces that push the body up from the braced position into ball impact and create longitudinal axis rotation in the arm. In the normally functioning kinetic chain, the legs and trunk segments are the motor for force development and the stable proximal base for distal mobility3,6,7. This compound develops 51-55% of the kinetic energy and force transferred to the hand7, generates the angular momentum from hind limb to fore limb to propel the arm forward8,9, and has large mass and height due to its large cross-sectional area Moment of inertia creates an anchor that allows centripetal motion2,3. The functional result of this stable base is considered core stability10. When core stability and its power and torque generating capability are not being utilized, other, less efficient methods must be used to maintain optimal performance. This is difficult due to the smaller size of the remaining muscles. Mathematical analysis has shown that a 20% decrease in trunk kinetic energy requires a 33% increase in shoulder speed or a 70% increase in shoulder mass to maintain the same kinetic energy at the hand the majority of the force8, decreases the internal Rotational torques at the shoulder11, creates greater muscle forces at the shoulder8, allows more shoulder abduction to produce top spin, reduces bounce12, and produces greater racquet and ball speeds13,14. This type of activation is the most efficient and is more commonly seen in elite male players. Figures 1 to 3 show electromyogram activation patterns in the lower limb characteristic of the push-through pattern. Figure 1 (elite player) shows the progression of activation in the gastrocnemius and quadriceps muscles from hind leg to front leg before ball impact14; whereas Figures 2 and 3 show activation of the hamstrings and gluteus maximus prior to ball impact15.
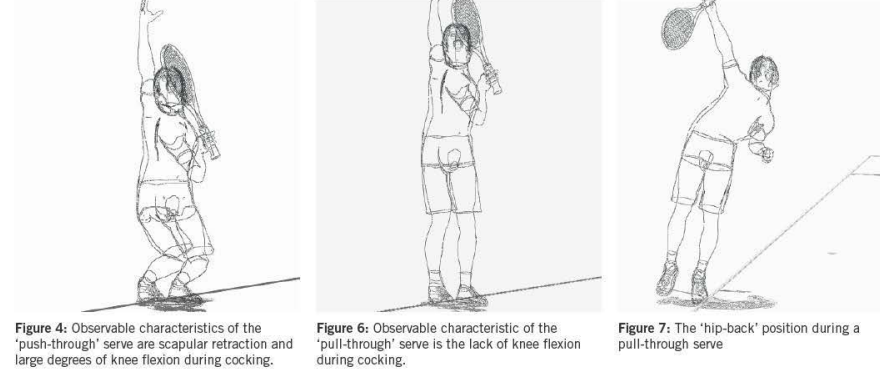
Figure 4 shows observational characteristics of push-through activations. The player’s knees are bent, the rear hip tilts back and counter-rotates away from the field; The torso does not overextend during contraction and the arm is in line with the shoulder blade and torso. Pull-through activation uses core muscles to pull the core and arm from instep into ball impact, creating longitudinal axis rotation in the arm. Knee flexion and use of the legs are minimized. This activation increases internal rotational torques at the shoulder8, creates increased scapular protraction and glenohumeral hyperangulation16, decreases shoulder abduction and ability to hit topspin12, and is associated with reduced ball speeds14. This type of activation results from a lack of full utilization of the proximal kinetic chain segments and is more common in female elite and recreational players.
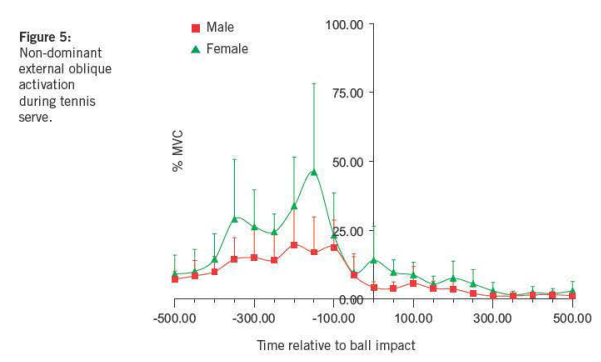
SUMMARY
Tennis players must use their kinetic chains to move their body and arm through ball impact. The push-through technique is more efficient and associated with better usability. Observation can distinguish push-through from pull-through, and strategies can be employed to improve the tennis player’s ability to develop the push-through kinetic chain.
References:
- Putnam CA. Sequential motions of body segments in striking and throwing skills: descriptions and explanations. J Biomech 1993; 26: 125-135.
- Cordo PJ, Nashner LM. Properties of postural adjustments associated with rapid arm movements. J Neurophysiol 1982; 47: 287-308.
- Zattara M, Bouisset S. Posturo-kinetic organization during the early phase of voluntary upper limb movement. J Neurol Neurosurg Psychiatry 1988; 51: 956-965.
- Nieminen H, Niemi J, Takala EP. Load sharing patterns in the shoulder during isometric flexion tasks. J Biomech 1995; 28: 555-566.
- Marshall R, Elliott BC. Long axis rotation: the missing link in proximal to distal segmental sequencing. J Sports Sci 2000; 18: 247-254.
- Elliott B. The development of racquet speed. In: Elliott B, Reid M, Crespo M, eds. Biomechanics of advanced tennis. London: International Tennis Federation 2003. p. 33-47.
- Kibler WB. Biomechanical analysis of the shoulder during tennis activities. Clin Sports Med 1995; 14: 79-86.
- Fleisig GS, Nicholls R, Elliot BC, Escamilla RF. Kinematics used by world class tennis players to produce high-velocity serves. Sports Biomech 2002; 2: 51-71.
- Elliott BC, Marshall R, Noffal G. Contributions of upper limb segment rotations during the power serve in tennis. J Appl Biomech 1995; 11: 443-447.
- Kibler WB, Press J, Sciascia AD. The role of core stability in athletic function. Sports Medicine 2006; 36: 1-11.
- Elliot BC, Fleisig GS, Nicholl R, Escamilla RF. Technique effects on upper limb loading in the tennis serve. J Sci Med Sport 2003;6: 76-87.
- Bahamonde R, Knudson D. Linear and angular momentum in stroke production. In: Elliott B, Reid M, Crespo M, eds. Biomechanics of advanced tennis. London: International Tennis Federation 2003. p. 49-70.
- Bahamonde R. Changes in angular momentum during the tennis serve. J Sports Sci 2000; 18: 579-592.
- Girard O, Micallef JP, Millet GP. Lower-limb activity during the power serve in tennis: effects of performance level. Med Sci Sports Exerc 2005; 37: 1021-1029.
- Lintner D, Noonan TJ, Kibler WB. Injury patterns and biomechanics of the athlete’s shoulder. Clin Sports Med 2008; 27: 527-552.
- Pink MM, Perry J. Biomechanics of the shoulder. In: Jobe FW, ed. Operative techniques in upper extremity sports injuries. St Louis: Mosby 1996. p. 109-23.
- Burkhart SS, Morgan CD, Kibler WB. The disabled throwing shoulder: spectrum of pathology Part I: pathoanatomy and biomechanics. Arthroscopy 2003; 19: 404-420.
- Lukasiewicz AC, McClure P, Michener L. Comparison of three dimensional scapular position and orientation between subjects with and without shoulder impingement. J Orthop Sports Phys Ther 1999;29: 574-586.